Convulsiones Neonatales
WHEC Boletín Práctica Clínica y Gestión de los proveedores de salud. Educación subvención prevista de Salud de la Mujer y el Centro de Educación (WHEC).
Neonatal seizures are an important example of an age-specific seizure syndrome. Compared with seizures at older ages, neonatal seizures differ in etiology, semiology, and electro-encephalographic (EEG) signature, and can be refractory to antiepileptic drugs that are effective in other age populations. Their unique pathophysiology has become the subject of many research studies from a basic and clinical perspective and is leading the way to new therapies for this often-refractory disorder. After delivery at term (37 – 41 weeks of gestation), neonatal seizures, an indicator of neurologic dysfunction, are associated with the need for resuscitative efforts and hypothermia therapy and are linked with epilepsy and cerebral palsy. Notably, a component of the definition of neonatal encephalopathy is seizure activity in the earliest days of life. Currently used therapies have limited efficacy.
The purpose of this document is to provide an overview of current approaches to the diagnosis and treatment of neonatal seizures, identifying some of the recent insights about the pathophysiology of neonatal seizures that may provide the foundation for better treatment. The lifespan of seizures is highest in the neonatal period. Although the treatment of neonatal seizures has not significantly changed in the last several decades, there has been substantial progress in understanding developmental mechanisms that influence seizure generation and responsiveness to anticonvulsants.
Prevalence
The reported incidence of neonatal seizures varies widely. The risk of seizures is highest in the neonatal period (1.8 – 5/1,000 live births in the US). The relative incidence is higher in premature infants less than 30 weeks gestation, occurring in 3.9% of these neonates compared with 1.5% of older infants (1). In the neonate, a broad range of systematic and central nervous system (CNS) disorders can increase the risk of seizures. Most neonatal seizures are symptomatic; they can be extremely difficult to control with currently available antiepileptic drugs (AEDs) and can lead to long-term neurologic sequelae. Benign forms include benign familial neonatal seizures and transient, treatable metabolic derangements; these forms are largely without significant long-term consequences.
The most common cause of symptomatic neonatal seizures is hypoxic/ischemic encephalopathy (HIE), with affects approximately 1 -2/1,000 live births (2). In fact, about two-thirds of cases of neonatal seizures are due to HIE (2). These seizures can occur in the setting of birth asphyxia, respiratory distress, or as a complication of early life extracorporeal membrane oxygenation (ECMO) or cardiopulmonary bypass for corrective cardiac surgery. In the case of HIE, these seizures usually occur within the first 1 - 2 days of birth and often remit after a few says but carry with them a risk of long-term epilepsy and neurological/cognitive deficits (3). HIE is associated with a high incidence of seizures, reportedly in 40 - 60% of cases (3).
Based on clinical observation, seizures are thought to occur in approximately 0.5% of term newborns and 20% of preterm newborns. However, long-term EEG recording in pharmacologically paralyzed term newborn suggest that the incidence may be as high as 40% (4).
Definition
A seizure is defined generally as an excessive, synchronous electrical discharge (i.e., depolarization of neurons in the brain). However, this definition may be too restrictive when applied to seizures in the newborn. Neonatal seizures may be defined more aptly as paroxysmal alterations in neurologic function (e.g., behavioral, motor, or autonomic function). This definition encompasses both clinical phenomenon that correlate temporally with epileptiform EEG abnormalities and stereotypical, paroxysmal clinical activities that are not associated clearly with EEG alterations (4).
Etiologies of Neonatal Seizures
Many pathologies can give rise to a neonatal seizures (stated below), hypoxic-ischemic (HIE) insults ("asphyxia") account for about nearly 50% of cases of neonatal seizures. Very few neonatal seizures are idiopathic about 2 - 5% (5).
Acute Metabolic (5%): Hypoglycemia; Hypocalcemia; Hypomagnesemia; Hypo- or hyper natremia; Withdrawal syndromes associated with maternal drug use (4%); Iatrogenic associated with inadvertent fetal administration of local anesthetic; Rare inborn errors of metabolism (including pyridoxine responsive). Two rare syndromes, early myoclonic encephalopathy (EME) and early infantile epileptic encephalopathy (EIEE), characteristically present in the first weeks with severe recurrent seizures and are associated with inborn errors of metabolism. Both lead to severe subsequent neurodevelopmental impairment. During the neonatal period, the seizures are fragmentary myoclonic, and clonic in EME, and tonic in EIEE, both showing a burst-suppression EEG pattern.
Cerebrovascular: Hypoxic ischemic encephalopathy (HIE) (46%); Arterial and venous ischemic stroke; Intra-cerebral hemorrhage; Intra-ventricular hemorrhage (10%); Sub-dural hemorrhage; Sub-arachnoid hemorrhage; Cerebral artery infarction (6%).
Central Nervous System (CNS) Infection (17%)s: Bacterial meningitis; Viral meningoencephalitis; Intrauterine ("TORCH") infections.
Developmental: Multiple forms of cerebral dysgenesis (4%).
Other: Rare genetic syndromic disorders; Benign neonatal familial convulsions (sodium and potassium channel mutations identified); Early myoclonic encephalopathy; Neonatal epileptic syndromes; Idiopathic (2%).
Types of Clinical Seizure
A clinical seizure is a sudden, paroxysmal depolarization of a group of neurons that results in a transient alteration in neurological state. This may involve abnormal motor, sensory, or autonomic activities, with or without a change in conscious level (6). Neonatal seizures differ in clinical description from those in adults, and seizures in preterm infants differ from those in term infants. Cerebral cortical organization, synaptogenesis, and myelination of cortical efferent pathways are poorly developed in human neonates, leading to weakly propagated, fragmentary seizures whose electrical activity may not spread to surface EEG electrodes. There are four recognizable clinical seizure types (7): Subtle; Clonic; Tonic; and Myoclonic; (See table 1 below).
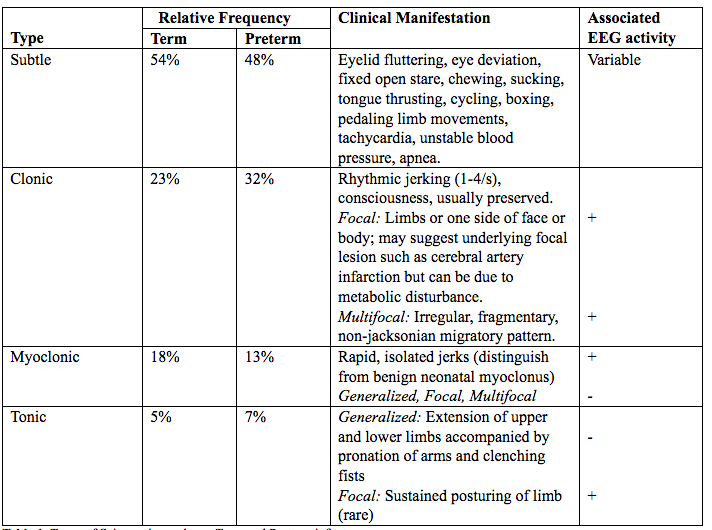
Table 1. Types of Seizures in newborn: Term and Preterm infants
Pathophysiology
The neonatal period is a period of intense physiological synaptic excitability, as synaptogenesis occurring at this time point is wholly dependent upon activity. In the human, synapse and dendritic spine density are both peaking around term gestation and into the first month of life (8). In addition, the balance between excitatory versus inhibitory synapses is tipped in the favor of excitation to permit robust activity-dependent synaptic formation, plasticity, and remodeling (9). Glutamate is the major excitatory neurotransmitter in the CNS, while γ-amino-butyric acid (GABA) is the major inhibitory neurotransmitter. There is considerable and growing evidence from animal models and human tissue studies that neurotransmitter receptors are highly developmentally regulated (See Figure 1 below) (9,10).
Studies of cell morphology, myelination, metabolism and more recently neurotransmitter receptor expression suggest that the first 1-2 weeks of life in the rodent is a roughly analogous stage to the human neonatal brain. Equivalent developmental periods are displayed for rats and humans on the top and bottom x-axes, respectively.
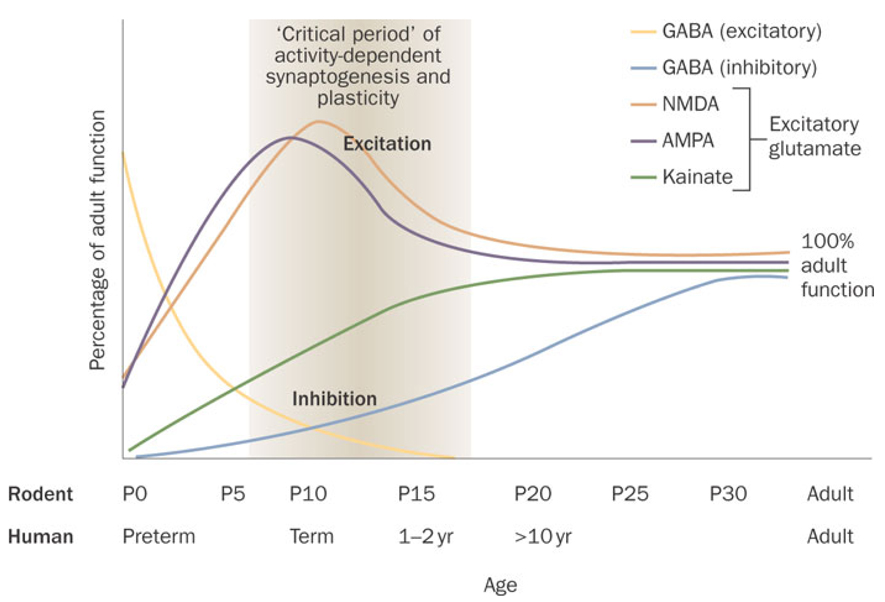
Figure 1. Schematic depiction of developmental profile of glutamate and GABA receptor expression and function. Abbreviations: GABA (γ-amino-butyric acid); NMDA (N-methyl-D-aspartate); AMPA (α-amino-3-hydroxy-5-methyl-4-isoxazoleproprionic acid)
Activation of GABA receptors is depolarizing in rats early in the first postnatal week and in humans up to and including the neonatal period. Functional inhibition, however, is gradually reached over development in rats and humans. Prior to full maturation of GABA-medicated inhibition, the NMDA (N-methyl-D-aspartate) and AMPA (α-amino-3-hydroxy-5-methyl-4-isoxazoleproprionic acid) subtypes of glutamate receptors peak between the first and second postnatal weeks in rats and in the neonatal periods in human. Kainite receptor binding is initially low and gradually rises to adult levels by the fourth postnatal week. Neonatal seizures emerge within the "critical period" of synaptogenesis and cerebral development.
Enhanced excitability of the neonatal brain: Depicted in figure 2, are an excitatory glutamatergic synapse (left panel) and a GABAergic inhibitory synapse (right panel). Presynaptic release of glutamate results in depolarization (excitation) of the postsynaptic neuron (left panel) by activation of NMDA and AMPA receptors. In contrast, release of GABA (right panel) results in hyperpolarization (inhibition) when the post synaptic neuron expresses sufficient quantities of the Cl (chloride) transporter KCC2, but depolarization (excitation) when intracellular Cl accumulates due to unopposed action of the Cl importer NKCC1.
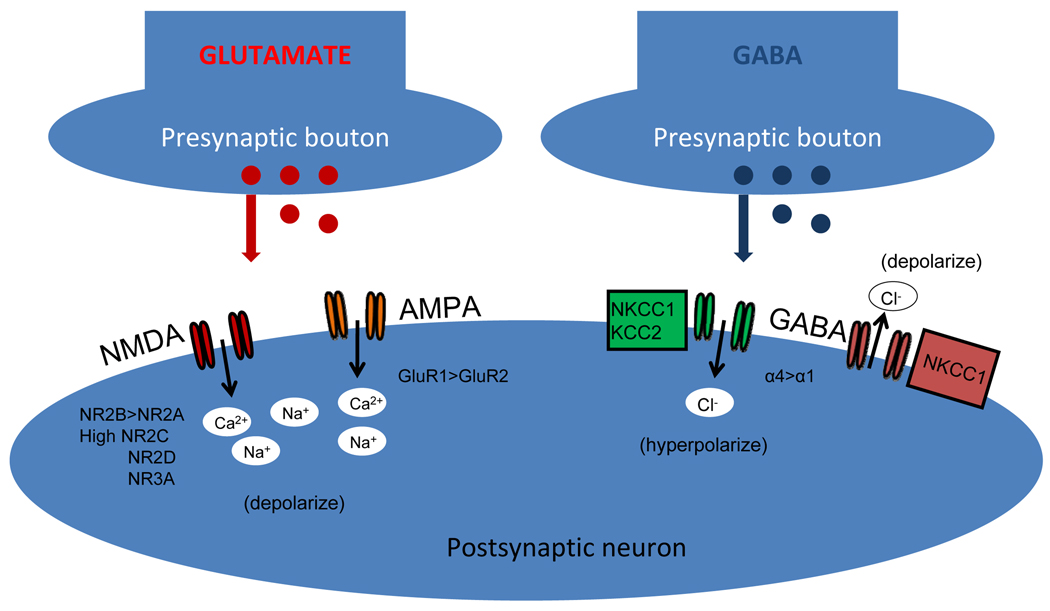
Figure 2. Dynamics of synaptic transmission at cortical synapses in the neonatal period.
Decreased efficiency of inhibitory neurotransmission in the immature brain: The immature glutamatergic receptors (left panel figure 2) are comprised of higher levels of NR2B, NR2C, NR2D, and NR3A subunits of the NMDA receptor, enhancing influx of Ca2+ and Na+ compared to mature synapses. In addition, AMPA receptors are relatively deficient in GluR2 subunits, resulting in relatively increased Ca2+ permeability compared to mature synapses. Hence specific NMDA receptor antagonists and AMPA receptor antagonists may prove to be age-specific therapeutic targets for treatment development. In addition, while GABAA receptor activation normally results in hyperpolarization and inhibition at mature synapses, due to the co-expression of NKCC1 and KCC2, the expression of KCC is low in the neonatal period compared with later in life and thus Cl levels accumulate intracellularly and opening of GABAA receptors allows the passive efflux of Cl out of cell, resulting in paradoxical depolarization (11).
Ion channel configuration favors depolarization in early life: Ion channels also regulate neuronal excitability, and like neurotransmitter receptors, are developmentally regulated. Mutations in the K+ channels KCNQ2 and KCNQ3 are associated with benign familial convulsions (12). These mutations interfere with the normal hyperpolarizing K+ current that prevents repetitive action potential firing. Hence, at the time when there is an overexpression on GluRs and incomplete network inhibition, a compensatory mechanism is not available in these mutations. Another K+-channel super-family member, the HCN (or h) channels, is also developmentally regulated. The h currents are important for maintenance of resting membrane potential and dendritic excitability, and function is regulated by isoform expression (13).
A role for neuropeptides in the hyperexcitability of the immature brain: Neuropeptide systems are also dynamically fluctuating in the perinatal period. An important example is corticotropin releasing hormone (CRH), with elicits potent neuronal excitation (14). Compared with later life, the perinatal period CRH and its receptors are expressed at higher levels, specifically in the first 2 postnatal weeks in rats. CRH levels increase during stress, and thus seizure activity in the immature brain may exacerbate subsequent seizure activity. Notably, adrenocorticotropic hormone, which has demonstrated efficacy in infantile spasms, also known to downregulate CRH gene expression (15). Hence, neuropeptide modulation may be an area of future clinical importance in developing novel neonatal seizure treatment.
Enhanced potential for inflammatory response to seizures in the immature brain: Neonatal seizures can occur in the setting of inflammation either due to an intercurrent infection or secondary to hypoxic/ischemic injury. Experimental and clinical evidence exists for early microglial activation and inflammatory cytokine production in the developing brain in both hypoxia/ischemia, and inflammation. Importantly, microglia have been shown to be highly expressed in immature white matter in rodents and humans during cortical development (16). During normal development as well as in response to injury, microglia participate in "synaptic stripping" by detaching presynaptic terminals from neurons. Importantly, the microglial inactivators minocycline and doxycycline have been shown to be protective against seizure-induced neuronal death and also protective in neonatal stroke models.
Selective neuronal injury in the developing brain: While many studies suggest that seizures, or status epilepticus, induce less death in the immature brain than in the adult, there is evidence that some neuronal populations are vulnerable. Similar to the sensitivity of subplate neurons, hippocampal neurons in the perinatal rodent have been shown to undergo selective cell death as well as oxidative stress following chemo-convulsant-induced cell death. These neurons are critical for the normal maturation of cortical networks. Importantly, in both humans and rodents these cells possess high levels of both AMPARs and NMDARs (17). In addition, these cells may also lack oxidative stress defenses present in mature neurons. A number of studies have shown that the application of clinically available antioxidants, such as erythropoetin (Epo), is protective against neuronal injury in neonatal stroke. Recently, Epo was shown to decrease later increases in seizure susceptibility of hippocampal neurons following hypoxia-induced neonatal seizures in rats (18).
Seizure-induced neuronal network dysfunction: potential interaction between epileptogenesis and development of neurocognitive disability: Given that there is minimal neuronal death in most models of neonatal seizures, the long-term outcome of neonatal seizures is thought to be due to seizure-induced alterations in surviving networks of neurons. Evidence of this theory comes from several studies that reveal disordered synaptic plasticity and impaired long-term potentiation as well as impaired learning later following brief neonatal seizures. The neonatal period represents a stage of naturally enhanced synaptic plasticity when learning occurs at a rapid pace (19).
Diagnosis and Investigations
Neonatal seizures can be difficult to diagnose as there are often no clinical correlates of the electrographic seizures, a phenomenon called electroclinical dissociation. Infants can show no signs or very subtle tonic or clonic movements, often limited to only one limb, making the diagnosis difficult to discern from myoclonus or other automatisms. A recent study revealed that approximately 80% of EEG-documented seizures were not accompanied by observable clinical seizures (20). The timing of seizures can be helpful indicator, such as in the case of "fifth day fits," due to hypocalcemia. Pyridoxine-dependent seizures present as refractory early neonatal seizures that uniquely respond to parenteral pyridoxine administration. Seizures that continue to be refractory in the setting of a history consistent with HIE manifest within first 24-48 hours of life; persist over several days, then appear gradually to remit.
The large differential diagnosis following a neonatal seizure (Table 1) demands that any investigations should be undertaken in a logical sequence. Certain clues to the etiology may be present, such as a history of perinatal asphyxia or maternal narcotic abuse, but other causes such as hypoglycemia, hypocalcemia, and CNS infection may co-exist and need excluding.
First Line of Investigations
Pulse oximetry;
Glucose levels;
Packed cell volume;
Serum calcium (ionized if possible), sodium, magnesium levels;
Arterial pH;
Lumbar puncture, blood cultures;
Cranial ultrasound scan;
Electroencephalogram (EEG).
Second Line of Investigations
MRI (magnetic resonance imaging) or CT (computed tomogram), Magnetic resonance spectroscopy;
Maternal and neonatal samples (urine/hair/meconium) for drug abuse;
Specimens for virology and congenital infections;
Serum ammonia, amino acids;
Urinary amino acids and organic acids;
Consider therapeutic trial or pyridoxine.
Imaging
Cranial ultrasound scanning is readily available in most centers, and it is useful as a first line imaging investigation for exclusion of gross CNS pathology, CNS malformations, periventricular hemorrhage, but it may fail to detect other forms of clinically important pathology, such as cerebral arterial infarction, subdural and subarachnoid hemorrhage. If the initial ultrasound examination is normal but the infant continues to have seizures or has abnormal interictal neurological signs, a CT or MRI examination should be carried out.
MRI can provide important information in terms of cerebral dysgenesis and gross structural malformations which can be associated with neonatal seizures such as tuberous sclerosis, hemimegaloencephaly, or cortical dysplasia. For symptomatic seizures due to HIE, abnormal T2, FLAIR and diffusion signals can be used to pinpoint regional injury and severity (21). Recent studies show that magnetic resonance spectroscopy can be used to predict severity and outcome in patients.
Electroencephalogram (EEG)
EEG is essential for diagnosis and for assessing treatment efficacy. Full 20-lead EEGs are most sensitive in detection these often-multifocal seizures (Figure 3). As full-lead EEGs can be difficult to obtain on an emergent basis in many neonatal intensive care units (NICU), amplitude-integrated EEG (aEEG) devices are becoming increasingly utilized. aEEG is usually obtained from a pair or limited number of leads and is displayed as a fast Fourier spectrum transform.
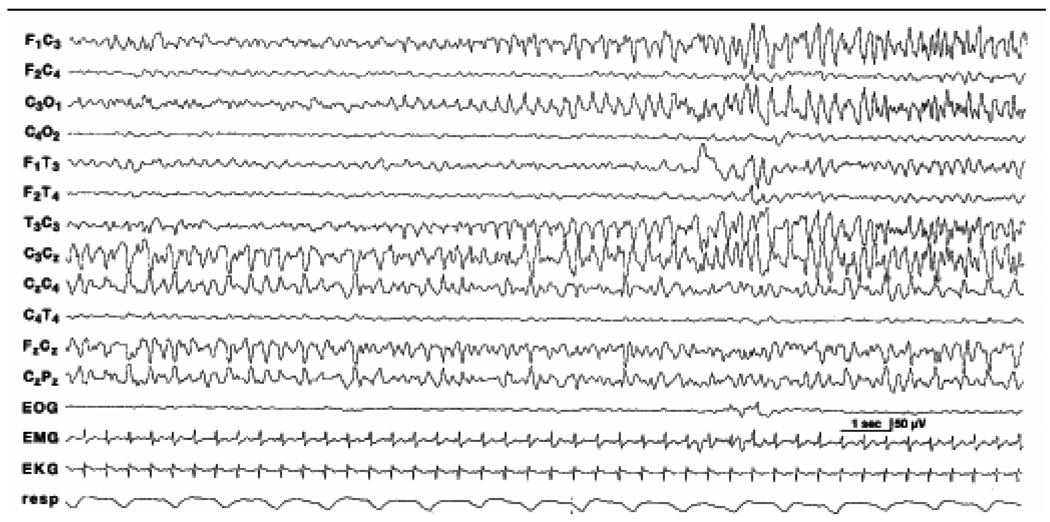
Figure 3. Electroencephalographic (EEG) appearance of neonatal seizure. Electric seizure activity begins in the midline central region (CZ) and then shifts to the left central region (C3). Toward the end of seizures, as the electrical activity persists in the left central region, the midline region becomes uninvolved. This electrical seizure activity occurred in the absence of any clinical seizures activity in this 40-week gestational age female infant with hypoxic-ischemic encephalopathy (HIE). She was initially comatose and hypotonic, and the time of EEG recording, had been treated with phenobarbital.
With aEEG, seizures are detected by acute alterations in spectral width, and a raw EEG from the single channel can be accessed by the viewer for confirmation (22). Several reports now indicate that aEEG has relatively high specificity by compromised sensitivity, detecting approximately 75% of that of conventional full lead montage EEG (23).
Continuous EEG recording is recommended for infants who are paralyzed to detect the frequency and duration of seizures. Interictal background EEG abnormalities are helpful in determining prognosis in both term and preterm infants. A poor prognosis is associated with burst-suppression and persistent low voltage states, although experience is required when assessing EEGs of preterm infants, as these normally show discontinuous activity with long interburst intervals.
Treatment
Two principles exist. The first is to detect and treat the underlying cause of the seizure, paying particular attention to associated acute metabolic disturbances, such as hypoglycemia. The second principle is the assessment of the need to control the seizures, which involves balancing the benefits of stopping some or all of the seizures against any potential deleterious effects of anticonvulsant medication. It is this second principle that provokes the greatest debate.
Pharmacologic treatment options for neonatal seizures have expanded over the last two decades. Treatment algorithm from the review of the literature and available data is shown in table 2. Phenobarbital and phenytoin/fosphenytoin have traditionally been the most commonly used medications to treat neonatal seizures, despite only approximately 50% efficacy (24). Phenobarbital is the first-line treatment given in neonatal seizures. There is extremely limited evidence on the effect of phenobarbital on long-term neonatal development. Although phenytoin was found to be equally effective, the potential for side-effects, the unpredictability of metabolism in neonates, and the need for frequent blood-level monitoring prevent us from recommending it as a first-line option.
Levetiracetam, phenytoin/fosphenytoin, and lidocaine all appear potentially effective as second-line treatments for neonatal seizures that are unresponsive to phenobarbital. Based on our systematic review findings alone, there is not strong evidence to recommend the use of any one of the medications over the others for second-line seizure control. Levetiracetam appears to acutely reduce seizure burden in many studies (26). It does not require blood-level monitoring, is easily continued as outpatient therapy, and is one of the few FDA-approved antiepileptics for children as young as one month of age. When used, we suggest dosing of 40 - 50 mg/kg bolus in line, which shows good rapidity of response without any significant side effects.
Lidocaine appears effective compared to benzodiazepines but has a narrow therapeutic window and the potential to cause cardiac arrhythmias or hypotension and can induce seizures at high doses (25). Lidocaine should also not be given following fosphenytoin/phenytoin because they may have additive cardiodepressive effects. Phenytoin/fosphenytoin was shown to only provide about a 10 - 15% increase in seizure control when given following phenobarbital failure (25). It also requires frequent blood-level monitoring and is not an ideal medication for maintenance at discharge given its erratic oral absorption, frequency of dosing (usually every 6 - 8 hours) and containing drug metabolism changes in young infants. Blood loss due to therapeutic drug monitoring may be benign in older patients but is potentially harmful and increases the risk of transfusion and associated morbidities/mortality in neonates whose blood volumes average 80 ml/kg.
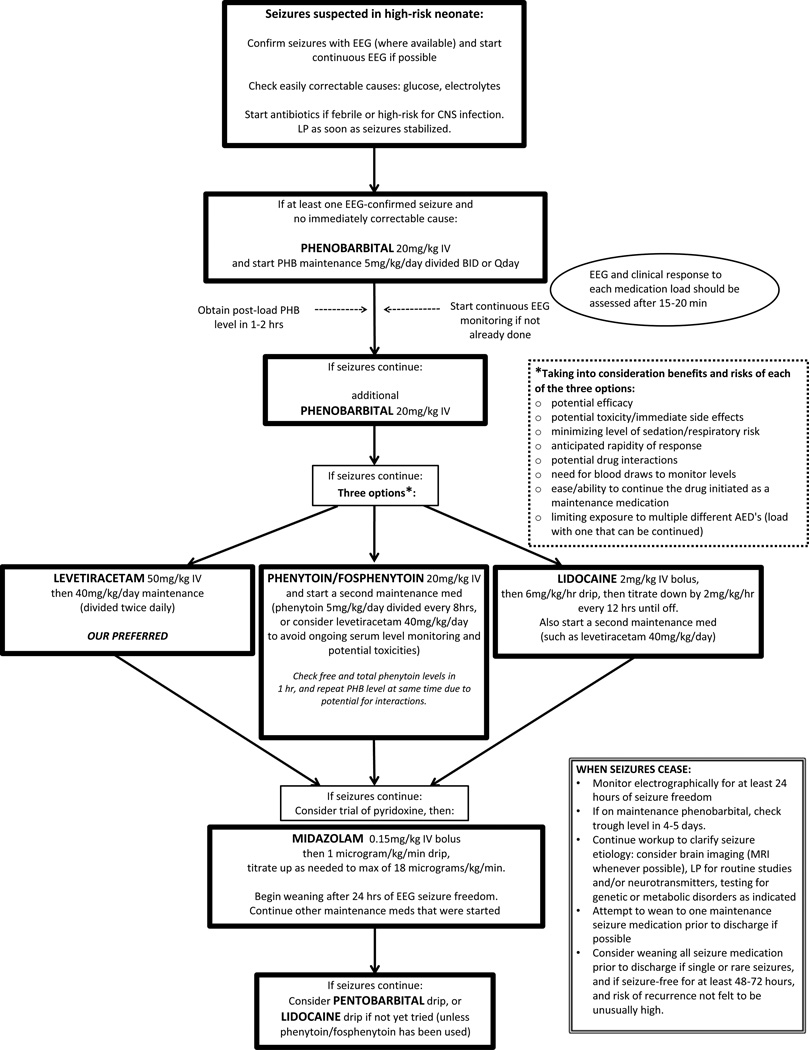
Table 2. Suggested treatment algorithm for recurrent neonatal seizures. Solid arrow indicates next step if electrographically-confirmed seizures are continuing (clinical or subclinical). Abbreviations: CSF (cerebrospinal fluid); CNS (central nervous system); EEG (electroencephalogram); IV (intravenous); LP (lumbar puncture); MRI (magnetic resonance imaging); PHB (phenobarbital)
A serious side-effect of benzodiazepines including that of midazolam is sedation, potentially leading to respiratory depression and intubation. Still, midazolam may be considered as second--or third-line therapy choice, especially in already intubated neonates.
Neonatal seizures can be extremely refractory to conventional AEDs, especially those associated with HIE. Early diagnosis should isolate metabolic or infectious etiologies and direct care to correcting the primary cause. However, the most refractory seizures are those due to asphyxia (repetitive) and due to their short course (over 72-96 hours) and poor prognosis, early treatment is essential and should be guided by EEG documentation of seizure activity. Current practices include early treatment with phenobarbital (doses ranging from 20-40 mg/kg), with phenytoin (20 mg/kg), or fosphenytoin, and/or benzodiazepines such as lorazepam (0.05 - 0.1 mg/kg) as second line adjuvant therapy for refractory seizures (7). However, consensus is that currently used AEDs are often ineffective for treatment of neonatal seizures (27). Indeed, phenobarbital and phenytoin appear to be equally but incompletely effective, and either drug alone controls seizures in fewer than half of EEG-confirmed neonatal seizures. As an alternative, second-line treatment with midazolam has variable efficacy, yet is less of a respiratory depressant. Lidocaine may be effective in refractory neonatal seizures, but its use may be limited by potential cardiac toxicity. Newer AEDs such as topiramate and levetiracetam have been anecdotally reported to improve acute neonatal seizures. It is also not known how long to continue treatment following the short course of neonatal seizures, and how the length of treatment impacts outcome (28).
In addition to pharmacological therapy, neonates with HIE are also being increasingly treated with hypothermia. Recent clinical studies have led to a Cochrane review endorsement that early whole-body or limited cranial hypothermia improves neurologic outcome in treated neonates. For whole-body hypothermia, current practice is to decrease core body temperature to 33.5o C for 72 hours (6). While aEEG is routinely employed to monitor brain activity during hypothermia, the effect of hypothermia on the incidence or severity of neonatal seizures is yet to be determined (29).
Levetiracetam vs. Phenobarbital for Neonatal Seizures
There are no US Food and Drug Administration (FDA) approved therapies for neonatal seizures. Phenobarbital and phenytoin frequently fail to control seizures. There are concerns about safety of seizure medications on the developing brain. Levetiracetam has been proven efficacy and an excellent safety profile in older patients; therefore, there is great interest in its use in neonates. However, randomized studies have not been performed. This study evaluated efficacy and safety of levetiracetam compared with phenobarbital as a first-line treatment of neonatal seizures (34). The primary outcome measure was complete seizure freedom for 24 hours, assessed by independent review of the EEGs by 2 neurophysiologists.
Conclusion of this phase IIb study was, phenobarbital was more effective than levetiracetam for the treatment of neonatal seizures. Higher rates of adverse effects were seen with phenobarbital treatment. Higher-dose studies of levetiracetam are warranted, and definitive studies with long-term outcome measures are needed (34).
Long-Term Prognosis with Neonatal Seizures
Poor prognosis for premature infants with seizures is reflected in high rates of subsequent long-term disability and mortality. The severity and timing of the pathologic process continue to be the major determinants for outcome (30). The prognosis is determined primarily by etiology. The prognosis following isolated hypocalcemia or benign familial neonatal seizures is excellent. Early hypoglycemia, CNS infections, and hypoxic-ischemia have and intermediate prognosis (30-50% normal), while CNS malformations are generally associated with a poor neuro-developmental outcome.
Seizure characteristics associated with adverse outcome are generalized myoclonic or tonic seizures, interactable seizures, and burst-suppression or persistent low voltage EEG states. A normal interictal EEG is associated with a good outcome. Other clinical factors associated with poor outcomes are persisting neurological abnormalities on clinical examination and very low birth-weight. Only 25% of infants less than 31 weeks of gestation had a normal outcome after EEG confirmed seizures, compared with 60% of term infants (31). Children with neonatal seizures but who subsequently were considered to have a normal outcome were followed up in their late teenage years and found to have difficulties with spelling, arithmetic and memory, despite having normal overall intelligence (7). This suggests that the underlying CNS vulnerability which contributes to neonatal seizures may be of longer-term clinical importance.
Although neonatal seizures after delivery at term in low-risk pregnancies occur infrequently, they are associated with adverse outcomes in neonatal-maternal dyads, including increased rate of both neonatal and infant mortality (32). The results are useful for counseling and informing clinicians about the rate of adverse outcomes and a potential nidus for planning an intervention trial to mitigate associated morbidity and mortality.
Epilepsy, Febrile Seizures and Subsequent Risk of Attention-Deficit/Hyperactivity Disorder (ADHD)
Epilepsy, febrile seizures, and attention-deficit/hyperactivity disorder (ADHD) are disorders of the central nervous system. This study indicated a strong association between epilepsy in childhood, and to a lesser extent, febrile seizure and subsequent development of ADHD, even after adjusting for socioeconomic and perinatal risk factors, and family history of epilepsy, febrile seizures, or psychiatric disorders (33). The population prevalence of epilepsy has been estimated to be 0.5% to 0.9% worldwide, with the highest incidence rate found in very early childhood. Low gestational age, birth weight, and Apgar score are associated with an increased risk of epilepsy and febrile seizures. These risk factors have also been associated with an increased risk of ADHD. Other common risk factors include parental age, family history, and genetic factors, and this scenario has led to hypothesis of a common pathophysiological link between epilepsy and psychiatric disorders in general, and ADHD in particular. Children with febrile seizures have a 30% increased risk of ADHD (33).
Early identification of high-risk groups may improve their outcome. Among patients with epilepsy, comorbid neurodevelopmental disorders are highly prevalent, including ADHD. Several hypotheses have been suggested for the association between seizures and ADHD. Previous studies of children with ADHD without seizures have found increased rates of pathologic findings on EEG. Common environmental risk factors for neurodevelopment vulnerability predisposing children to both disorders have also been proposed. Common genetic risk factors for both disorders could also be an explanation. Finally, severity of the convulsions may influence the risk of developing ADHD, as may the nature of febrile seizures (simple versus complex). Identifying groups of children at high risk of ADHD is important because having this disorder is known to increase use of health services and the risk of injuries, substance abuse disorder, criminally, psychotic disorders, and premature death. It is important for healthcare providers to identify early symptoms of ADHD in patients with epilepsy and febrile seizure, to initiate proper assessment and treatment, and thereby reduce the likelihood of negative long-term consequences of ADHD.
Summary
Seizures in the newborn are different from those occurring in older children and adults. Healthcare professionals may find it difficult to recognize neonatal seizures because their sometimes-subtle manifestations. Conclusive behavior has many etiologies; some are associated with a favorable and some with a poor prognosis. Treatment protocol is controversial because both the seizures themselves and the drugs used to treat them may damage the immature brain. The high mortality and morbidity rates of infants who experience seizures make it imperative that the neonatal seizure be recognized, its etiology diagnosed, and treatment begun promptly. There is limited evidence regarding the best pharmacologic treatment for neonatal seizures. We anticipate that this review of neonatal seizure treatment will serve as a valuable reference that will aid healthcare providers and inform the design for future investigations to compare the effectiveness of neonatal antileptics. Further research is needed not only to determine which antiepileptic medication is most effective in neonates, but also to verify the safety of treating neonates with these medications.
References
- Jensen FE. Neonatal seizures: an update on mechanisms and management. Clin Perionatol 2009;36(4):881-900
- Tekgul H, Gauvevreau K, Soul J, et al. The current etiologic profile and neurodevelopment outcome of seizures in term newborn infants. Pediatrics 2006;117(4):1270-1280
- Ronen GM, Buckley D, Penny S, Streiner DL. Long-term prognosis is children with neonatal seizures: a population-based study. Neurology 2007;69(19):1816-1822
- Hill A. Neonatal seizures. Pediatrics 2000;21(4):117-121
- World Health Organization. Guidelines on neonatal seizures. Geneva 2011; Available at: https://www.ncbi.nlm.nih.gov/books/NBK304092/ Last retrieved on 22 May 2021
- Shankaran S, Laptook AR, Ehrenkranz RA, et al. Whole-body hypothermia for neonates with hypoxic-ischemic encephalopathy. N Engl J Med 2005;353(15):1574-1584
- Volpe JJ. Neurology of the newborn. 5th edition. Philadelphia: Saunders/Elsevier; 2008
- Haynes RL, Bornstein NS, DeSilva TM, et al. Axonal development in the cerebral white matter of the human fetus and infant. J Comp Neurol 2005;484(2):156-167
- Rakhade SN, Jensen FE. Epileptogenesis in the immature brain: emerging mechanisms. Nat Rev Neurol 2009;5(7):380-391
- Sanchez RM, Jensen FE. Maturational aspects of epilepsy mechanisms and consequences for the immature brain. Epilepsia 200142(5):577-585
- Dzhala VI, Talos DM, Sdrulla DA, et al. NKCC1 transporter facilitates seizures in the developing brain. Nat Med 2005;11(11):1205-1213
- Cooper EC, Jan LY. M-channels: neurological diseases, neuromodulation, and drug development. Arch Neurol 2003;60(4):496-500
- Yue C, Yaari Y. KCNQ/M channels control spike after depolarization and burst generation in hippocampal neurons. J Neurosci 2004;24(19):461-424
- Ju WK, Kim KY, Neurfeld AH. Increased activity of cyclooxygenase-2 signals early neurodegenerative events in the rats retina following transient ischemia. Exp Eye Res 2003;77(2):137-145
- Brunson KL, Khan N, Eghbal-Ahamdi M, Baram TZ. Corticotropin (ACTH) acts directly on amygdala neurons to down-regulate corticotropin-releasing hormone gene expression. Ann Neurol 2001;49(3):304-312
- Billiards SS, Haynes RL, Folkerth RD, et al. Development of microglia in the cerebral white matter of human fetus and infant. J Comp Neurol 2006;497(2):199-208
- Talos DM, Fishman RE, Park H, et al. Developmental regulation of alpha-amino-3-hyroxy-5-methyl-4-isoxazole-propionic acid receptor subunit expression in forebrain and relationship to regional susceptibility to hypoxic/ischemic injury. J Comp Neurol 2006;497(1):42-60
- Gonzalez FF, McQuillen P, Mu D, et al. Erythropoietin enhances long-erm neuroprotection and neurogenesis in neonatal stroke. Dev Neurosci 2007;29(4-5):321-330
- Maffei A, Turrigiano G. The age of plasticity: Developmental regulation of synaptic plasticity in neocortical microcircuits. Prog Brain Res 2008;167:211-223
- Clancy RR. Prolonged electroencephalogram monitoring for seizures and their treatment. Clin Perinatol 2006;33(3):649-665
- Grant PE, Yu D. Acute injury to the immature brain with hypoxia with our without hypoperfusion. Radiol Clin North Am 2006;44(1):63-77
- Lawrence R, Mathur R, Nguyen The Tich S, et al. A pilot study of continuous limited-channel aEEG in term infants with encephalopathy. J Pediatr 2009;154(6):835-841.e1
- Clancy RR. Summary proceedings from the neurology group on neonatal seizures. Pediatrics 2006;117(3 Pt 2): S23-S27
- Slaughter LS, Patel AD, Slaughter JL. Pharmacological treatment of neonatal seizures: A systematic review. J Child Neurol 2013;28(3):351-364
- van Rooij LG, Toet MC, Rademaker KM, et al. Cardiac arrythmias in neonates receiving lidocaine as anticonvulsive treatment. Eur J Pediatr 2004;163(11):637-641
- Khan O, Chang E, Cipriani C, et al. Use of intravenous levetiracetam for management of acute seizures in neonates. Pediatr Neurol 2011;44(4):265-269
- Booth D, Evans DJ. Anticonvulsants for neonates with seizures. Cochrane Database Syst Rev 2004;18(4):CD004218
- Bartha AI, Shen J, Katz KH, et al. Neonatal seizures: multicenter variability in current treatment practices. Pediatr Neurol 2007;37(2):85-90
- Azzopardi D, Brocklehurst P, Edwards D, The TOBY Study Group, et al. The TOBY Study. Whole body hypothermia for the treatment of perinatal asphyxia encephalopathy: a randomized controlled trial. BMC Pediatr 2008;30(8):17
- Ronen GM, Buckley D, Penny S, Streiner DL. Long-term prognosis in children with neonatal seizures: A population-Based Study. Neurology 2007;69(19):1816-1822
- American College of Obstetrics and Gynecology. Task Force on Neonatal Encephalopathy; American Academy of Pediatrics. Neonatal encephalopathy and neurologic outcome. 2nd edition. Washington D.C.: American College of Obstetricians and Gynecologists; 2014
- Morgen SD, Chen HY, Chauhan SP. Neonatal seizures among low-risk pregnancies at term. Obstet Gynecol 2020;135:1417-1425
- Bertelsen EN, Larsen JT, Petersen L, et al. Childhood epilepsy, febrile seizures, and subsequent risk of ADHD. Pediatrics 2016;138(2):e20154654
- Sharpe C. Reiner GE, Davis SL, Nespeca M, et al. Levetiracetam versus phenobarbital for neonatal seizures: a randomized controlled trial. Pediatrics 2020;145(6):e20193182. Correction: Pediatrics 2021;147(1):e2020036806
Publicado: 8 June 2021
Dedicated to Women's and Children's Well-being and Health Care Worldwide
www.womenshealthsection.com